
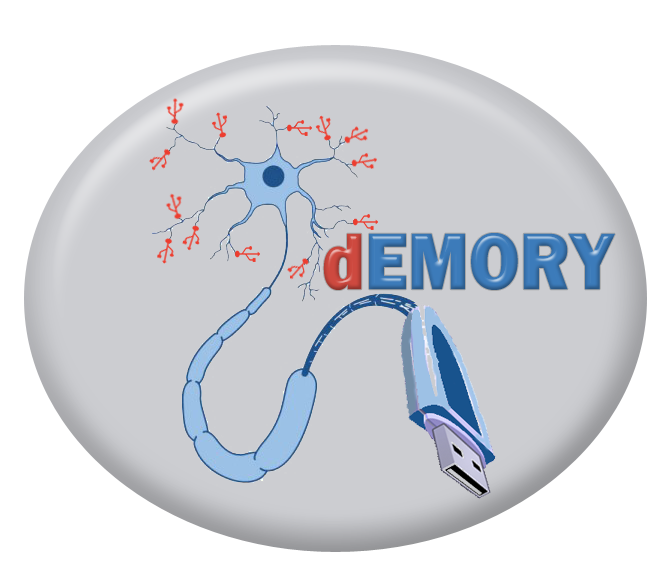
The purpose of this Research Topic is to compile the latest developments in Dendritogenesis, including new imaging techniques, the development of biosensors for dendrite activity detection, and to outline recent discoveries related to dendrite development and function in health or diseases. So far, several in vitro and in vivo model systems proved that various intrinsic and extrinsic parameters influence dendritogenesis, the dendritic electrical activity and its protein synthesis, but mechanisms of dendritogenesis are only partially understood. Numerous human diseases and disorders such as epilepsy are now known to be tied to dendritic function disruptions. Indeed, the formation of dendrites is essential for the development of neuronal circuit activity, but also for experience-dependent plasticity that plays a key role in physiological processes such as learning and memory. Understanding the development and regulation of dendritic arbors and spines, and the cell biological control of dendritic properties, presents an important and challenging field of research. Each dendrite possesses a unique arrangement of receptors and ion channels as well as organelles like mitochondria and ribosomes that enables it to alter protein density in response to changes in activity of neuronal inputs. Dendrites grow in length, enter a period of dynamic branching and retractions followed by a period of stabilization, where dendritic arbors are less dynamic. During development stages, the dendritogenesis relies on a combination of various factors including cell-intrinsic genetic programs and extracellular signals, such as trophic factors signaling, cell–cell interactions, and neuronal activity. Dendritic trees receive various synaptic inputs from other neurons and their primary function is to integrate and transmit these incoming signals to the cell body leading or not to the creation of an action potential. Dendritogenesis, the development of dendritic arbor, more precisely the number of dendrites, their shape and their branching pattern vary according to neuronal types. Most neurons fire in this way, but not all neurons are the same.Dendrites are specific extensive cytoplasmic regions that ensure effective connectivity in almost all neurons. This movement of a signal down a neuron is called an action potential. Once the electrical current reaches the end of the cell, it causes other chemicals to be released. These movements travel down the axon, like dominoes that have been tipped over.
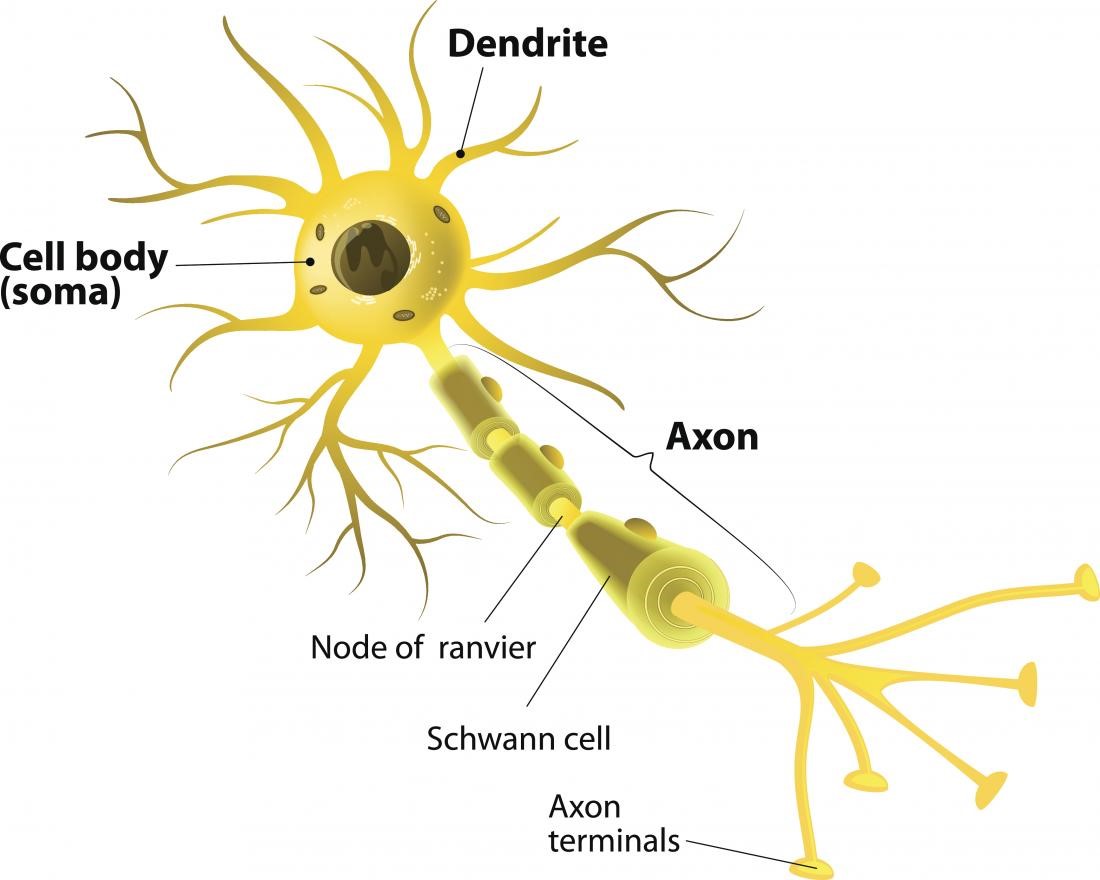
Ions, which are small, charged molecules, move in and out of entrances in the membrane. Then, the electrical nerve signal travels along an axon in a rush of chemistry. If the combination of neurotransmitters is correct, then they can cause an electrical current to sweep down the nerve cell. Nerve cells communicate with each other using chemicals called neurotransmitters. Nerve signals actually come down to some interesting chemistry. Let's look a bit closer at how this all works. As a final step, the signal leaves through the synapse to be passed along to the next nerve cell. Myelin sheaths cover the axon and work like insulation to help keep the electrical signal inside the cell, which makes it move more quickly. Next, the signal leaves the soma and travels down the axon to the synapse. From there, the signal travels to the main cell body, known as the soma. Neurons receive these signals from neighbor neurons through their dendrites. Other signals are instructions for your organs, glands and muscles. Some of these signals bring information to the brain from outside of your body, such as the things you see, hear, and smell. Neurons pass messages to each other using a special type of electrical signal. From there the message can move to the next neuron. The message then moves through the axon to the other end of the neuron, then to the tips of the axon and then into the space between neurons. Nerve Cell: Dendrites receive messages from other neurons.
